Exploring the Birth of Heavy Elements in the Universe
Written on
Chapter 1: The Formation of Heavy Elements
Researchers at CERN and Argonne National Laboratory are delving into the origins of heavy elements, such as gold, platinum, and uranium, believed to be produced from neutron star collisions in the depths of space.
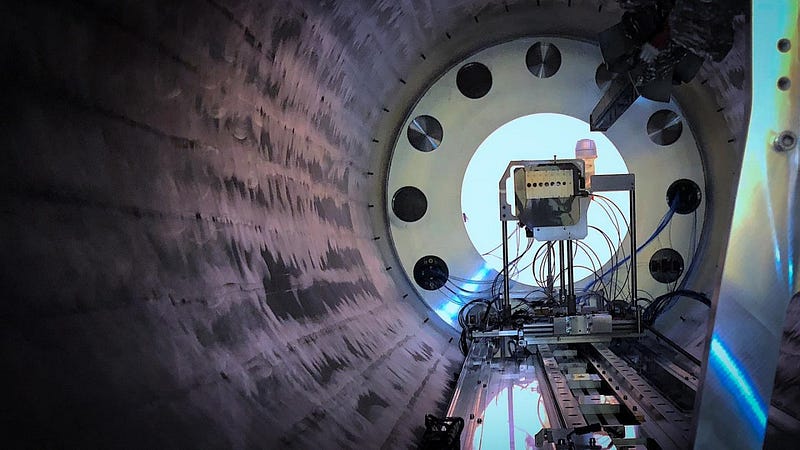
While scientists have a general understanding that elements are formed in the nuclear reactors at the core of stars, there remains uncertainty regarding the synthesis of the heaviest elements. Certain elements, including gold and uranium, are thought to require conditions even more extreme than those found in stars. Events such as neutron star mergers and colossal supernova explosions are prime candidates for these extreme conditions.
Last year marked a significant advance in this field when researchers discovered strontium, a heavy element, near a neutron star merger. Following this, Argonne National Laboratory scientists have brought their investigations to CERN, utilizing innovative techniques to explore the characteristics and formation processes of heavy elements. Their findings, recently published in Physical Review Letters, could reshape our understanding of stellar events and the early universe.
“One of the most pressing questions of this century has been how elements formed at the universe's inception,” remarks Ben Kay, a physicist at Argonne and the study's lead author. “Since we can't excavate a supernova, we must recreate these extreme environments to study the reactions that occur.”
During their research, the team made history by being the first to observe an atomic nucleus containing fewer protons than lead—82—and over 126 neutrons, known as “magic numbers” in nuclear physics.
Section 1.1: Understanding Magic Numbers
The established “magic numbers” in atomic nuclei are 8, 20, 28, 50, and 126. Nuclei at these numbers exhibit enhanced stability compared to others, akin to how noble gases achieve stability through complete electron shells.
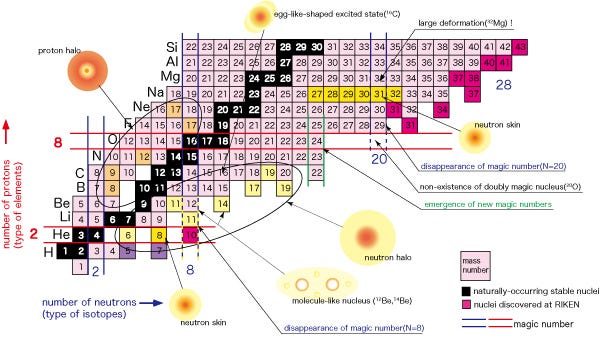
The region of the nuclear chart below 82 protons and above 126 neutrons remains largely uncharted, as producing these nuclei is exceptionally challenging. Investigating these nuclei is crucial for comprehending the rapid neutron-capture process, or r-process, believed to be responsible for creating many of the universe's heavy elements.
The r-process can occur in environments like neutron star mergers and supernovae, where there is an abundance of free neutrons. This allows for neutron capture, resulting in the formation of new, heavier elements. In standard conditions, these elements would decay before accumulating enough neutrons, but in a neutron-rich environment, the process accelerates.
To conduct their study, the researchers focused on the mercury isotope ²??Hg, which consists of 80 protons and 127 neutrons. They believe that studying this isotope could provide insights into the properties of neighboring nuclei in the region beyond the nuclear magic numbers, which are crucial for the rapid neutron-capture process.
Section 1.2: Conducting Experiments at CERN
To study ²??Hg, the team needed specialized equipment to generate a radioactive beam of mercury. Their experiment involved firing a high-energy beam of protons at a molten lead target, resulting in collisions that produce numerous exotic and radioactive isotopes. From these, the researchers extracted ²??Hg nuclei and directed them into a beam—reportedly the most energetic ever created at a nuclear accelerator facility—aimed at a deuterium target.
The experiment took place at the HIE-ISOLDE facility at CERN in Geneva, Switzerland, utilizing the deuterium target within the newly developed ISOLDE Solenoidal Spectrometer (ISS). This innovative magnetic spectrometer enabled the detection of instances where ²??Hg nuclei captured a neutron, transforming into ²??Hg.
“No other facility can create mercury beams of this mass and accelerate them to these energies,” Kay explains. “The exceptional resolving power of the ISS allowed us to observe the spectrum of excited states in ²??Hg for the first time.”
The key to artificially inducing neutron capture lies in the deuterium target, which is often referred to as “heavy hydrogen” due to its nucleus comprising a proton and a neutron. When ²??Hg captures a neutron from the deuterium target, the neutron separates from its proton partner, causing the proton to recoil. The emitted protons then hit the ISS detector, providing crucial information about the nucleus's structure and binding.
Chapter 2: Overcoming Experimental Challenges
Despite extensive studies into atomic nuclei, the exploration of heavy nuclei had previously faced challenges. The difficulty arises because while it is relatively straightforward to hit heavy targets with light ion beams, striking a light target with heavy ion beams is significantly more complex, leading to distorted physics that complicates analysis.
“When a heavy beam hits a delicate target, the kinematics change, and the resulting spectra become compressed,” Kay notes.
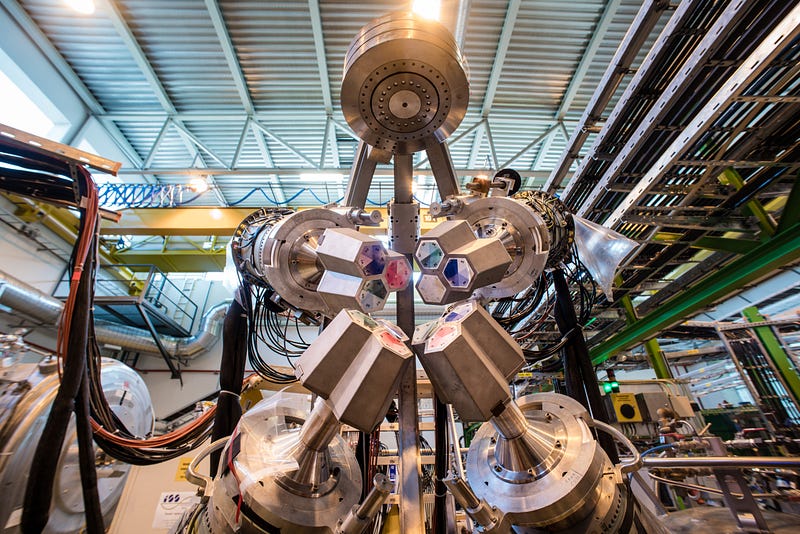
The team found a solution by utilizing the ISS, inspired by HELIOS, a groundbreaking concept introduced by Argonne distinguished fellow John Schiffer for the lab’s helical orbital spectrometer. Since 2008, HELIOS has enabled the exploration of nuclear properties previously deemed impossible. Building on this, CERN's ISOLDE facility can generate beams of nuclei that complement those produced at Argonne.
“John Schiffer realized that when collisions occur within a magnet, emitted protons travel in a spiral pattern towards the detector. By employing a mathematical ‘trick,’ this unfolds the kinematic compression, resulting in an uncompressed spectrum that reveals the underlying nuclear structure,” Kay concludes.
Initial results from the CERN experiments appear to validate the theoretical predictions made by current nuclear models. The research team plans to leverage the new capabilities and experimental techniques to investigate additional nuclei beyond the magic numbers, aiming for a deeper understanding of the universe's formation.
In the video "The Formation of Heavy Elements In The Beginning," experts discuss the processes that lead to the creation of heavy elements in the cosmos, providing valuable context for the ongoing research.
The video "Colliding Neutron Stars, Gravity Waves, and the Origin of the Heavy Elements" explores the role of neutron star collisions in the synthesis of heavy elements, complementing the findings of the research team.