Understanding Why Electric Motors Draw More Current When Stopped
Written on
Chapter 1: The Basics of Electric Motors
Let’s delve into an intriguing aspect of electric motors. Picture a small DC fan that is operational and spinning. At this point, if we measure the electric current flowing into the motor, we might observe a reading of 0.6 amps. However, once we halt the fan motor, the current surprisingly rises to about 1.0 amps. This phenomenon warrants investigation.
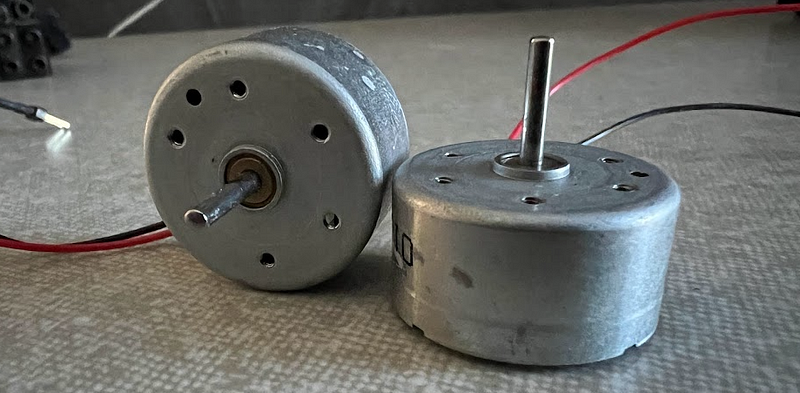
So, why does an electric motor consume more current when it is not in motion? To answer this, we must first understand what an electric motor is and how it operates. While there are various types of motors, our focus will be on the conventional DC motor with brushes.
A DC motor comprises a coil of wire carrying electric current and a permanent magnet. When the current flows through the coil, it generates a magnetic field. This magnetic field interacts with that of the permanent magnet, resulting in torque, which causes the coil to rotate in alignment with the external magnetic field.
To maintain the coil's rotation, two contact points are required. These points allow the battery's electric current to flow through the coil. As the coil turns, the wiring switches direction, reversing the current within the coil, thus sustaining the rotation. This mechanism is what we refer to as the "brush" system, where the battery wires function like tiny brushes sweeping over the moving coil.
Section 1.1: Simple DC Motors
Let’s examine a simplified version of an electric motor.
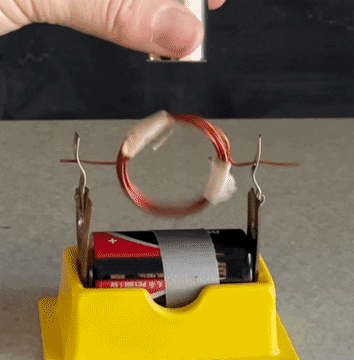
In this illustration, the coil of wire is coated with enamel to facilitate a current loop. The bottom half of the enamel on the two horizontal segments of the wire has been removed. This setup allows electrical contact with the supports in one position, enabling current flow. However, once it rotates to the enameled side, current ceases, yet it continues to rotate due to angular momentum. When it returns to the upright position, it receives another boost from the magnetic torque.
Subsection 1.1.1: The Transformation to Generators
If we detach the battery and connect the coil's ends to a light bulb instead, we can generate electric current. Spinning the coil in a magnetic field will induce voltage around the coil, resulting in current flow—effectively transforming it into an electric generator. However, this coil would require significant speed to produce noticeable results.
Here’s a related example: a stationary coil with a magnet moving in and out of it. A galvanometer indicates the direction of the induced current.
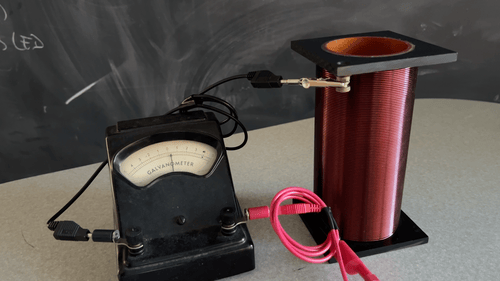
It's crucial to note that an induced electric current occurs only when the magnetic field within the coil is changing. This principle is articulated by Faraday’s Law, which we will explore further. Additionally, the induced current generates its own magnetic field that opposes the change in the external magnetic field, a concept that can be challenging to grasp.
Section 1.2: Exploring Faraday's Law
Now, let’s visualize a unique electric motor scenario. Imagine a coil of wire subjected to an electric current while positioned near a magnet.
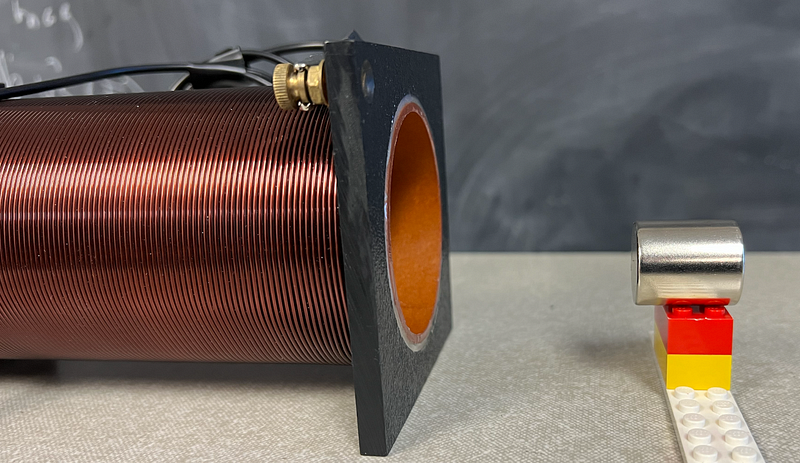
The current flowing through the coil produces a magnetic field that interacts with the magnet, creating an attractive force that pulls the coil toward it. As the coil moves closer, the magnetic field within it increases, which, according to Faraday’s law, induces a current that opposes this change. This results in a net current reduction.
On the other hand, if the same current flows through the coil while it remains stationary, the absence of a changing magnetic field means there’s no induced current in the opposite direction, leading to a higher current draw.
Let’s observe this phenomenon through a practical experiment. A hand-crank generator is connected to a battery, functioning as a typical electric motor.
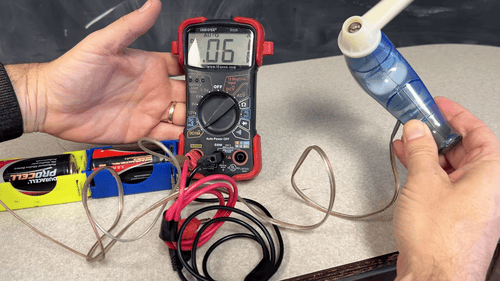
Notice two things: the current reading is positive, and the motor rotates clockwise. Now, if I remove the battery and manually crank the generator in the same direction, we can check the current reading again.
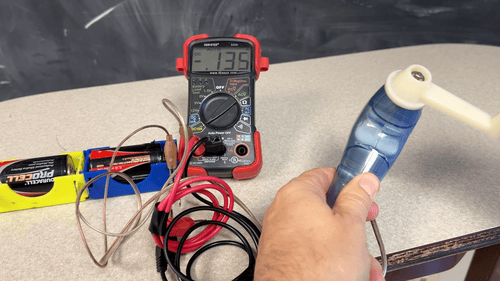
The ammeter indicates a negative current, which aligns with our expectations. This illustrates how an electric motor functions: it draws current to spin, while simultaneously acting as a generator that produces current in the opposite direction. The result is a reduced net current compared to a stationary coil.
Chapter 2: Implications for Electric Motors
Now, let’s apply this understanding to real-world examples. Consider a fan with an electric motor. If you stop the fan, not only will it halt air movement—likely the reason it was on in the first place—but the electric current will also surge. This increased current can cause the motor to overheat, especially since it no longer benefits from airflow for cooling.
In the case of a vacuum cleaner, which essentially functions as a fan within a casing, the situation is similar. If a sock gets lodged in the vacuum hose, you’ll notice a rise in the motor’s pitch, indicating that it spins faster due to reduced air resistance. While this increased speed leads to lower current consumption, the lack of air intake can again lead to overheating.
The first video titled "Why does an electric motor use more current when stopped? Physics." explains this phenomenon in further detail.
The second video, "Physics 12 U9L4 Electric Motors and Back EMF," provides additional insights into electric motors and their operation.