The Big Bang: Was It Really an Explosive Beginning?
Written on
Chapter 1: The Misconception of the Big Bang
When the term "Big Bang" is mentioned, what imagery does it evoke? Many envision a colossal fireball marking the universe's inception. Some might imagine a singularity akin to a black hole, suddenly erupting and flinging stars into space. Others may even picture the universe popping into existence with a satisfying "pop," reminiscent of a cork shooting from a champagne bottle. While these ideas are engaging, how accurate are they?
To understand the actual beginnings of our universe, we must journey back to the moment everything began. Prepare yourself, as this is truly the tale of everything.
At time zero, we encounter a challenge: the exact events of this moment remain largely unknown. However, we do have a few clues to guide us.
Section 1.1: The Nature of the Beginning
It’s essential to clarify that the universe did not originate from a black hole, despite what many sci-fi narratives suggest. If it had, we would expect to observe significant temperature variations throughout the cosmos, which we do not. Instead, the universe maintains a relatively uniform temperature, save for the occasional star.
Rather than being a singular explosive point, the Big Bang likely involved all of space being compressed into a minuscule ball—trillions of times smaller than a proton.
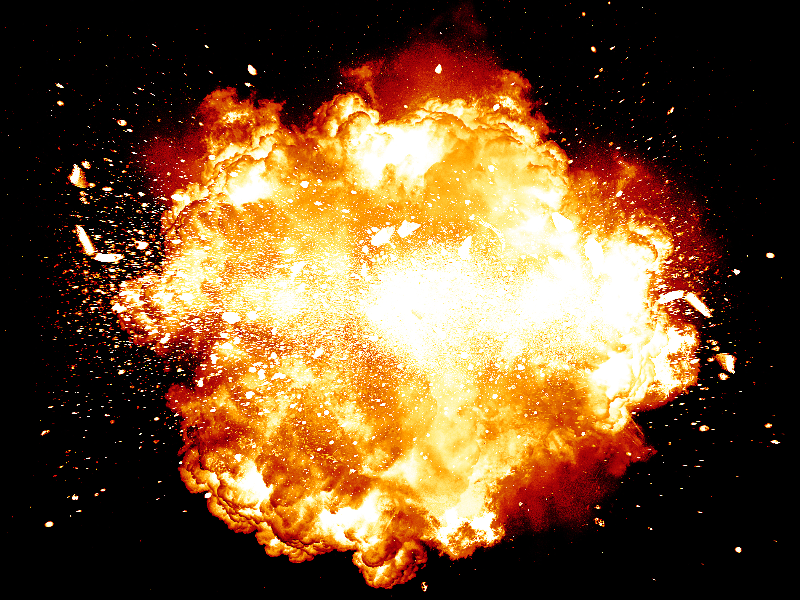
This early universe was not flat; it was, in fact, curved. If one could have paused time at this moment and walked straight ahead, they would have circled back to their starting point—much like the gameplay in classic Mario titles.
Moreover, there was no matter or distinct forces present. Instead, space was filled with a singular energy field, a concept that can be challenging to grasp.
In this primordial state, everything that constitutes our world—your body, the device you are currently using, your breakfast, the Solar System, and every galaxy—was squished into this infinitesimally small, curved universe.
Due to the extreme compression, everything existed as energy, allowing it to overlap in this tiny space. This energy wasn’t something we can observe today; it was an intrinsic energy field permeating the universe.
As there was no distinct matter, the four fundamental forces of nature—gravity, electromagnetism, the weak nuclear force, and the strong nuclear force—were unified into a single force. This unification occurred because there were no particles available to differentiate among them. Hence, all acted as one within this energy field.
Understanding these initial conditions requires a grasp of how Einstein's theory of General Relativity and Quantum Mechanics interrelate since both come into play at such a small scale.
Regrettably, we lack a comprehensive theory for Quantum Gravity at this point in time. Thus, our best understanding of the universe's beginnings is that it was a tiny sphere brimming with energy and governed by a singular force.
Section 1.2: The Expansion Begins
There’s another aspect to this 'ball' that deserves mention. The size of the universe remains uncertain, and it’s unclear whether it was truly curved. If we presume that the universe is as vast as the observable universe—currently estimated at 93 billion light-years across—imagine it being condensed into this minuscule sphere.
However, the entire universe is likely much larger than what we can observe and might even be infinite! So, even if the visible universe was compressed to the size of a proton, the true initial conditions could have been an infinitely large yet equally compact sphere.
But contemplating infinity and an infinitely large curved universe can be quite mind-bending! To simplify and avoid confusion, let’s focus on the observable universe as it existed in this tiny form at the beginning.
With the conditions set, are we about to witness a spectacular explosion?
Chapter 2: The Quark Era
At this stage, we arrive at a pivotal moment—the universe’s rapid expansion, often referred to as Inflation.
Within an astonishingly brief span—0.0000000000000000000000000000001 seconds—the universe transitioned from a ball the size of a proton to one the size of a football, becoming less curved and denser in the process.
The rapid expansion can be inferred from the uniform distribution of matter we observe today. Some scientists propose that the universe's initial curvature drove this swift expansion. As spacetime unfolded, it propelled the universe outward, flattening as it did so.
When we gaze into the cosmos today, we find that spacetime is predominantly flat; if you travel straight ahead, the universe's shape won’t distort your path—aside from the influence of gravity.
As the universe expanded and flattened, something remarkable occurred: the first fundamental particles emerged, initially in the form of Gluons, soon followed by Quarks.
With the universe's energy allowing for more space, particles began to condense out of it. Gluons, the carriers of the strong nuclear force, bonded Quarks together to form particles such as Protons, hence their name.
However, during this period, the universe remained too dense and hot for Quarks to combine into Protons. Instead, Gluons darted about freely!
It's noteworthy that the strong nuclear force has now separated from the original unified force. This is because the force-carrier particles, Gluons, have now become distinct from the energy field.
Once the universe expanded further, Gluons could combine to form Quarks and Antiquarks, which are attracted to one another. When they collide, they annihilate each other, releasing more Gluons.
This strange behavior illustrates the oddities of particle physics, where uniquely named particles engage in unusual interactions.
As Gluons represent energy and Quarks symbolize mass, the universe found itself balanced on the brink of 'mass equivalence.' Remember Einstein's famous equation E=MC²? It highlights that energy (E) and matter (M) are interchangeable. In this primordial phase, energy in the form of Gluons transitioned into matter in the form of Quarks, and vice versa. The universe oscillated between energy and matter.
At this juncture, no substantial matter existed; Quarks were fleeting and incapable of forming Protons or even atoms, meaning there was no medium for sound to propagate. Consequently, at this point, the universe was devoid of any explosive sound or even a faint whisper. Additionally, with the electromagnetic force still unified with the original force, there was no light to illuminate the darkness.
Thus, the universe remained silent and void of sound—a stark contrast to the explosion many might expect.
As events unfolded, an intriguing phenomenon occurred with Quarks and Antiquarks. While both exist in equal measure, our universe should have produced equal amounts of both. However, for every billion Antiquarks, one billion and one Quarks were formed. This slight surplus of Quarks would eventually account for all the matter in the universe, explaining its current emptiness.
Despite this fundamental particle warfare, no sound or light emerged from the chaos.
Chapter 3: The Natural Order Emerges
The universe is now a nanosecond old—a duration so brief that in this time, light travels just under 30 centimeters. Yet, despite its youth, the inflationary phase has dramatically progressed! The universe has expanded to a staggering size of one billion kilometers in diameter, roughly equivalent to the distance from Saturn to the Sun.
This rapid expansion allowed the universe to grow faster than the speed of light. This is permissible because it's the fabric of space itself that is expanding, not the objects within it. Thus, while Quarks and Gluons are constrained by light-speed travel, this rapid expansion adheres to the laws of physics.
If any curvature in spacetime existed previously, it has now flattened significantly. The cooling of the universe has reached a point where no new Quarks or Antiquarks are produced; the residual Quarks represent the total matter the universe will ever possess.
Consequently, we now observe a clear distinction between matter and energy. The fundamental forces of nature have finally separated from the original unified force, resulting in Gravity, Electromagnetism, Strong Nuclear Force, and Weak Nuclear Force acting independently on various Quarks.
With this separation, light can finally emerge as the Electromagnetic Force is now operational.
But where is our grand explosion?
Despite the formation of distinct particles, the universe remains too hot and dense to generate stable Protons or Neutrons (collectively known as Hadrons) or even smaller particles like Electrons (referred to as Leptons). Presently, the universe is merely a blend of Quarks, which do not constitute a stable form of matter, and thus sound cannot travel.
There was likely no light at this stage either—not in the bright, steady sense. While there may have been occasional Photons, a consistent glow was absent, resulting in a universe that remained dark and silent.
The Big Bang continues to be the quietest and most subdued explosion imaginable!
Chapter 4: Particles Begin to Form
As inflation continues, the universe expands from one billion kilometers to an impressive ten light-years in diameter. This substantial growth reduces the universe's density and temperature, setting the stage for the formation of the first stable particles.
At this point, one might wonder if we can finally witness or hear the Big Bang. Unfortunately, even with these particles forming, sound remains elusive. A medium is still lacking, as a sea of Hadrons does not constitute stable matter. While there may be fleeting Photons exchanged among particles, a distinct glow or flash remains absent.
Thus, the Big Bang remains a quiet, dim event.
Chapter 5: The Formation of Atomic Cores
By now, the universe has expanded to a diameter of 300 light-years, and the Hadrons and Leptons have cooled down. This cooling enables the next phase of atomic formation.
Protons are now cool enough to serve as the nuclei for Hydrogen, while Neutrons can combine with Protons to form nuclei of heavier isotopes like Hydrogen, Helium, and even Lithium. However, the temperature remains high enough that Electrons are still too energetic to bond with these nuclei, resulting in a state known as plasma—where the temperature is so elevated that Electrons are stripped from atomic nuclei.
The universe is now awash in a plasma primarily composed of Hydrogen, with a smattering of Helium and Lithium.
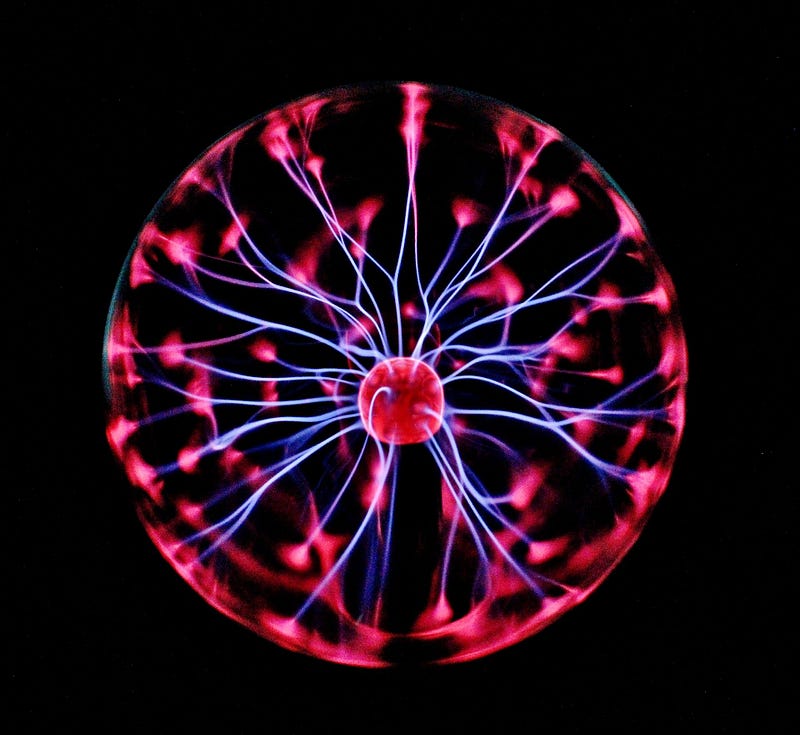
In this state, sound waves can finally propagate, but no significant events have occurred to generate them. The "sound" we could detect would merely stem from the temperature of the plasma, which reflects the average kinetic energy of the particles. The random movements of these nuclei colliding with one another would produce a very faint, white noise.
Though the plasma glows, it is not transparent. Consequently, the light is immediately reabsorbed and converted back into heat. This era is known as the Opaque Era—while there is an abundance of light, it cannot traverse the universe.
If one could peer into this universe, it would exhibit a glowing appearance, albeit with visibility limited by the plasma. The sound, too, would be a soft white noise, faintly perceptible only with specialized equipment.
Not quite the explosive spectacle we had anticipated!
Chapter 6: The Fog Lifts
This condition persists for approximately 379,000 years. During this time, the universe expands to around 42 million light-years, allowing the hot, dense plasma to cool to about 3000K (2726.85°C or 4940.33°F).
This cooling triggers a remarkable transformation: the plasma transitions to gas. Electrons no longer get knocked off atomic nuclei by heat, and they can finally bond with them to form the first true atoms. This marks the emergence of a transparent universe, as light no longer interacts with gas.
However, this transition did not occur instantaneously; it unfolded gradually over about 100,000 years as the plasma cooled and began to coalesce into gas.
To clarify, temperature is a measure of the average kinetic energy of a group of atoms. As the universe cooled, nuclei condensed into atoms, which were then bumped back into plasma as energy fluctuated. Over time, the ratio of atoms to nuclei increased, leading to the eventual formation of a sea of transparent gas.
After approximately 100,000 years (379,000 years post-Big Bang), no atom possessed sufficient energy to revert to plasma, resulting in a clear ocean of gas. However, no stars existed to illuminate this new realm, leading one to wonder what it would be like to observe it.
The light emitted by the hot plasma continued to fill the universe, but now it could travel freely without interacting with gas, as there were no solid objects to block or absorb the light.
The emitted light would resemble that of a tungsten bulb, as both emit light at similar temperatures.
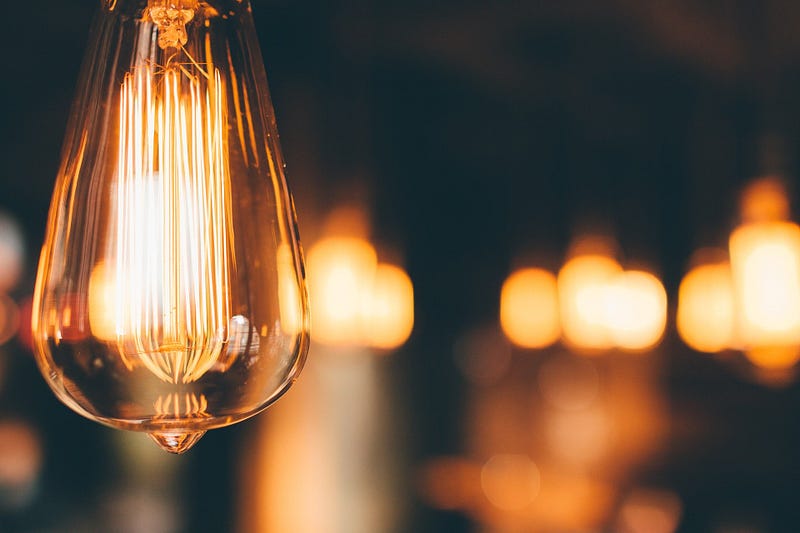
Today, we can still observe this primordial light, albeit stretched into a lower frequency due to the expansion of the universe. This phenomenon is known as the Cosmic Microwave Background (CMB), currently existing within the microwave spectrum rather than the visible light spectrum.
Thus, the fog gradually dissipated as the primordial plasma transitioned to transparency over 100,000 years. This wasn’t an explosion, but it did bring forth light! However, no real sound emerged, and the faint white noise previously detectable vanished, leaving behind a silence that persisted even with advanced audio equipment.
Chapter 7: The Universe Takes Shape
This marks the phase where the universe begins to take form. The slightly denser regions of primordial gas coalesce to form the first stars and galaxies. The explosive death of these stars leads to the creation of nearly all elements in the periodic table, along with planets and new stars.
One fortunate star gave rise to a third planet that turned out to be special—harboring life. This life eventually evolved into intelligent beings capable of experiencing emotions such as love, grief, and curiosity. These beings were capable of creating various artifacts, including spaceships, particle accelerators, art, and even biscuits.
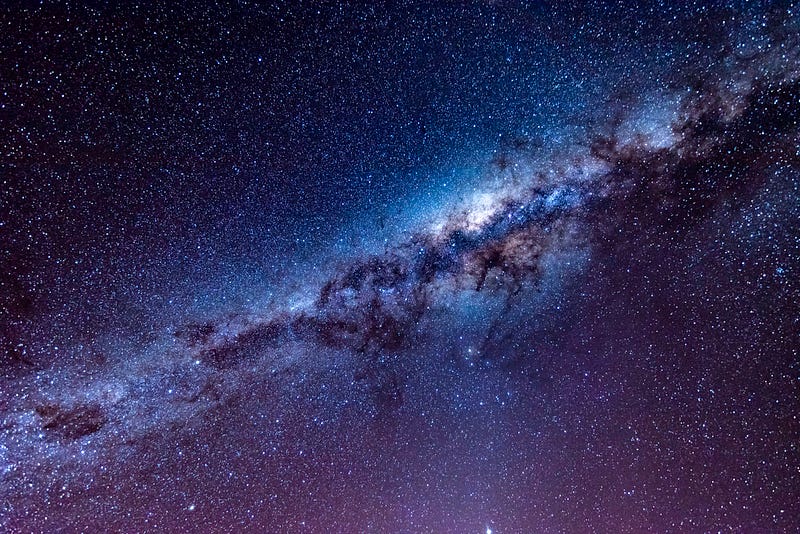
With this knowledge, we uncover the origins of everything. However, one lingering question remains: did we ever witness that explosive birth?
Conclusion: No Bang, No Problem
The explosive aspect of the Big Bang wasn't quite as explosive as many might envision. While it was indeed extremely hot and expanded at a rate surpassing light, the reality was more of a cosmic expansion of space rather than a conventional explosion.
The early universe was a unique environment, one devoid of structured matter and governed by unconventional physics. Additionally, our sensory perceptions are tailored for an atmosphere at cooler temperatures, making it impossible to truly hear or observe the birth of the universe.
If we could have "listened" to Quarks forming or "seen" spacetime unfolding, it might have resembled a tremendous explosion. Unfortunately, our limitations prevent us from experiencing it in that manner. Therefore, the universe's inception was dark and silent, followed by a soft glow and faint white noise.
Ultimately, there was no grand bang, no satisfying pop, and no rush of wind. Yet, this does not diminish the awe of our universe's origins.